
RESEARCH AREAS
Electrochemistry | Transport Phenomena | Catalysis | Ion-Conducting Polymers | Continuum Modeling
One of the great scientific feats that must be achieved this century is the deep decarbonization of our global economy. My work aims to use continuum simulations and electrochemical reaction engineering to assist in the development of cost-effective and efficient devices and materials that can address this challenge by using renewable energy to power the conversion of abundant feedstocks such as water, air, or carbon dioxide to the chemical commodities and fuels typically produced by unsustainable means. Additionally, leveraging electro-membrane separations, our group will develop technologies to valorize waste and remediate our atmosphere and oceans.
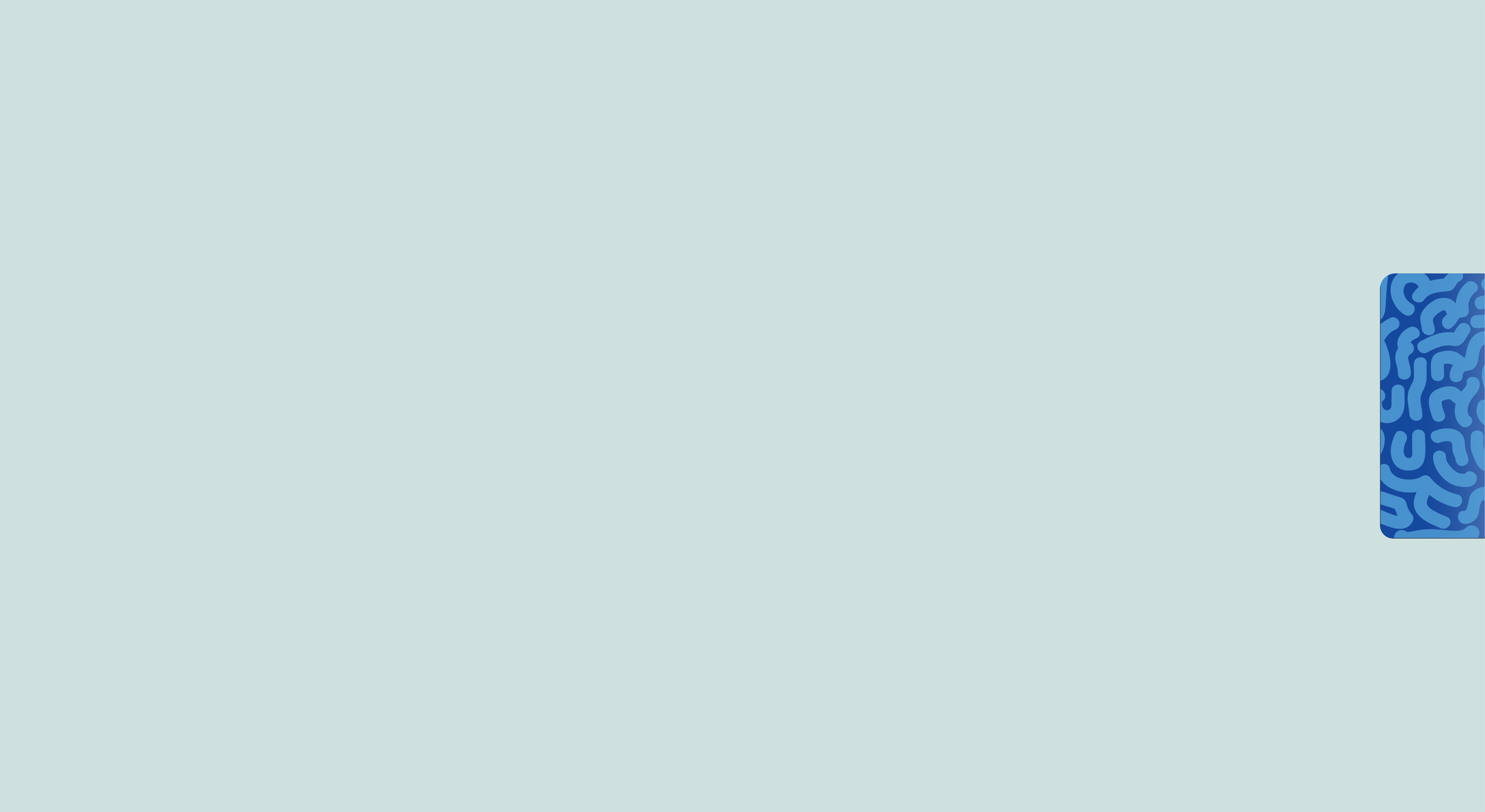
Bipolar membranes for electrosynthesis and environmental remediation
Due to their unique ability to maintain an applied pH gradient, BPMs hold immense potential to be an integral component in next generation devices for electrosynthesis and environmental remediation. By enabling the operation of a device under two-distinct microenvironments, BPMs facilitate optimal catalysis and mass transport for each of the individual half-reaction in an electrosynthesis architecture. Additionally, recent study has shown that the pH swings induced by BPMs can be used to liberate carbon dioxide from aqueous carbon capture solutions, or even to treat nitrogenous waste in municipal wastewaters. My research centers around implementing BPMs into electrochemical devices for industrial and environmental applications and resolving the role of multi-ion phenomena (salt-ion crossover, water dissociation, in situ CO2 generation) in dictating their behavior.
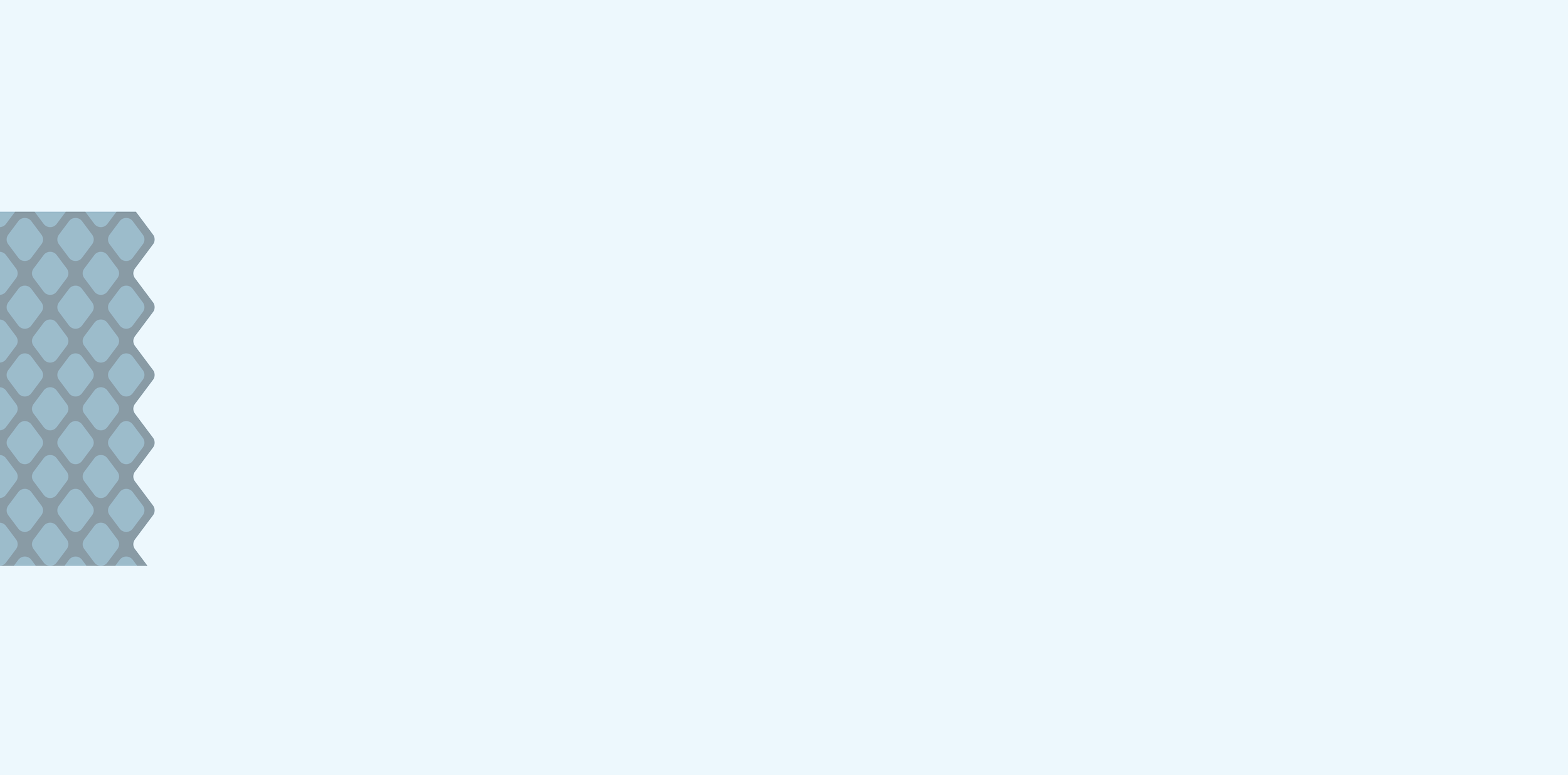
Multi-scale modeling of membrane-electrode assemblies
Membrane electrode assemblies (MEAs) will be ubiquitous reactor architectures in electrochemical synthesis and environmental remediation. However, the physics underpinning the transport of ions and the kinetics of catalytic reactions in these systems are still poorly understood. Leveraging porous electrode and concentrated solution theory to model the transport of species in these multi-phase systems at the mesoscale, along with quantum-mechanical theories of coupled-ion electron transfer to capture the kinetics of complex reaction chemistry, our group will develop “digital twins” of membrane electrode assemblies. These models will enable the acceleration of the design and optimization of these systems for industrial implementation of MEAs for chemical synthesis and environmental remediation.
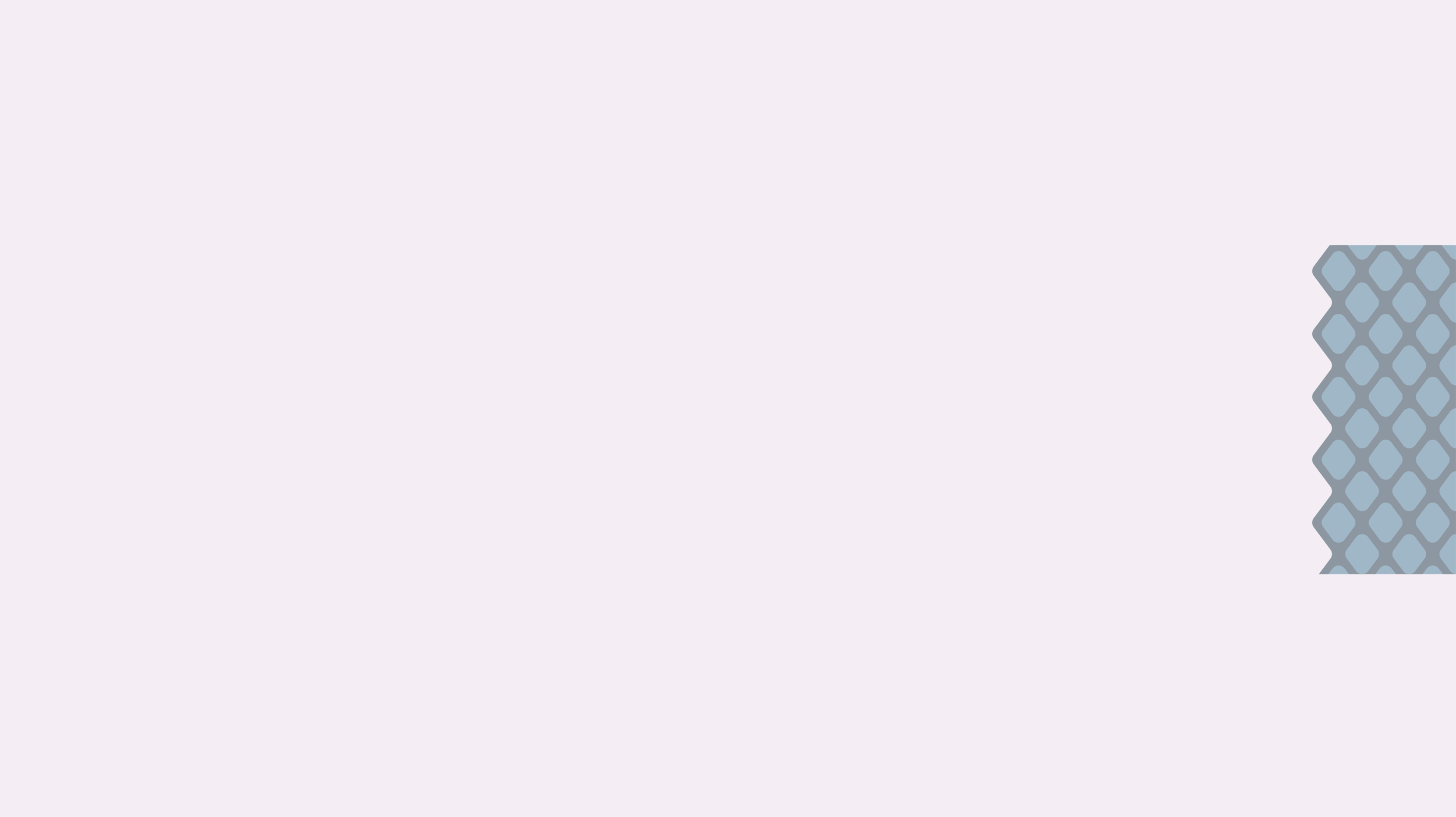
Devices for seawater electrolysis and carbon capture
The electrolysis of water to hydrogen fuel is a powerful technique to overcome the intermittency of current renewable energy technologies, and the ocean presents an untapped source of water for this chemical transformation. Unfortunately, standard water electrolyzers are intolerant of the impurities present in the ocean, and the evolution of chlorine gas at the anode presents a significant ecological concern for direct seawater electrolysis. Additionally, a substantial concern in carbon capture technologies is the fight against mass transfer for direct air capture, in which CO2 is only available at 400 ppm. Carbon species are one hundred times more concentrated in oceanwater, and recent work has shown electrochemical technologies can liberate CO2 from seawater. Leveraging my understanding of bipolar membranes and electrolysis devices, my research in this field focuses on developing cost-effective architectures capable of producing hydrogen or capturing carbon from the sea.
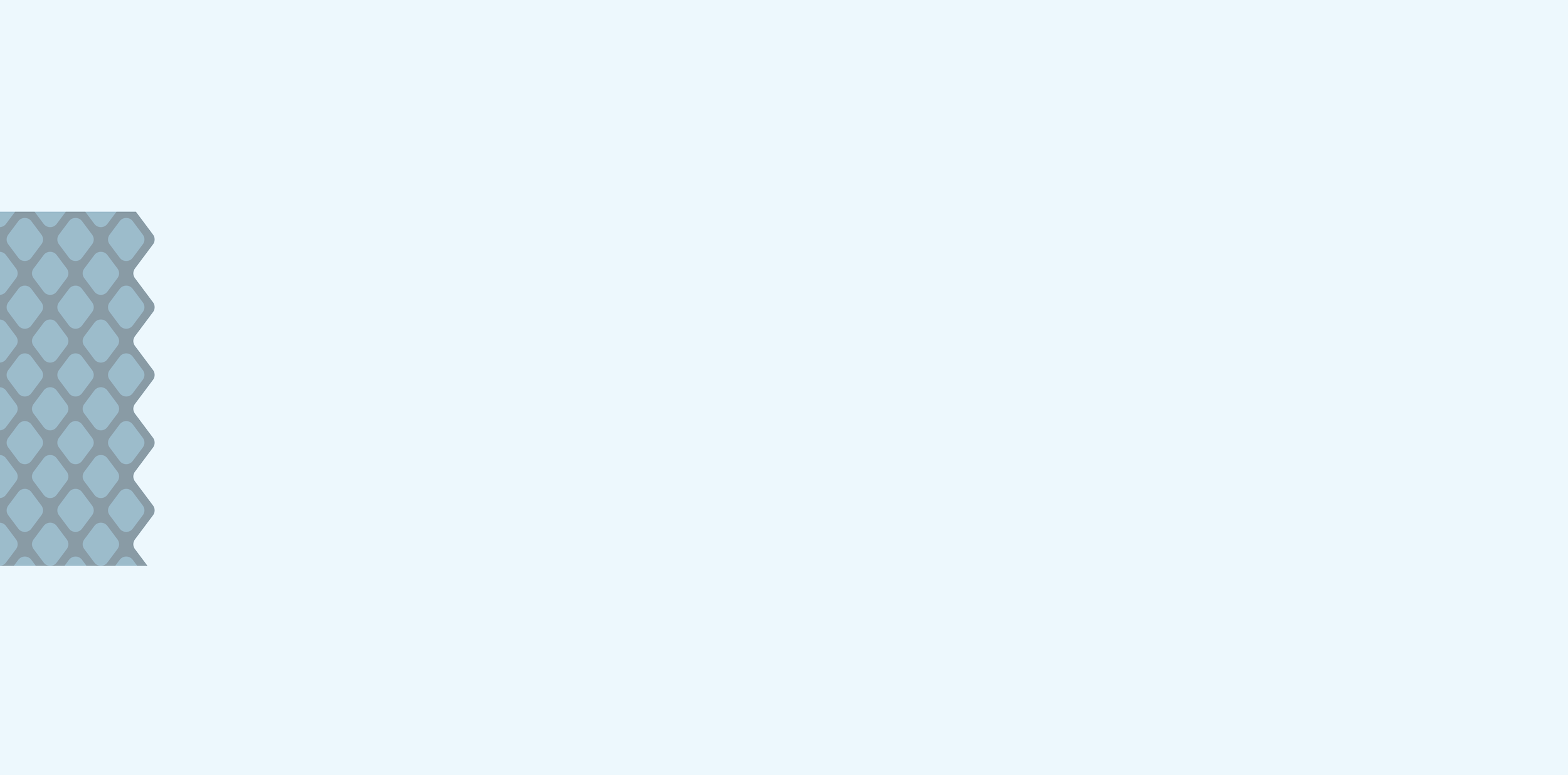
Advanced architectures for electrochemical synthesis
In recent years, electrochemical synthesis of hydrocarbon fuels or industrially relevant chemicals from abundant feedstocks (i.e., waste CO2, air, or water) has demonstrated the potential to mitigate greenhouse gas emissions and provide a method of storing and utilizing renewably sourced energy. Many electrochemical devices have been developed to perform electrochemical synthesis. However, most are limited in their application due to significant performance issues caused by low catalyst utilization, poor water management, poor selectivity to valuable products, and general efficiency losses. This work aims to evaluate a variety of advanced device architectures (membrane electrode assemblies, tailored catalyst environments, etc.) through a combination of experiments and multiphysics simulation to develop an electrochemical synthesis with optimal efficiency, selectivity, and lifetime. In particular, our group will explore the use of membrane-electrode assembly architectures for electrochemical oxygen atom transfers, CO2 electrolysis, and nitrogen reduction.